A primer on quantum computing storage and memory
Quantum storage and memory differ significantly from the technologies in today's computers. The implications for compute power and storage capacity could someday be extraordinary.
In 2017, Microsoft surprised attendees of its flagship Ignite conference when the company revealed its first quantum computer.
Since that time, quantum computing has been steadily gaining momentum, with several other technology providers building their own quantum computers. Storage administrators need to take note.
Even so, quantum computing is a work in progress, and there are still a number of hurdles for the technology to overcome. Many of the challenges pertain to memory and data storage.
The role of quantum memory in storage
Quantum computers cannot use conventional memory and data storage. They must instead use quantum memory, which is more capable than conventional memory but is also fragile and error-prone.
Quantum memory is based on quantum bits, or qubits. They're vastly different from the bits that conventional computers use. Typical computers use a system of binary bits that can have a value of either 0 or 1. Qubits can likewise store a 0 or 1, but thanks to a concept called superposition, they can also contain both at the same time. It is essentially a form of multidimensional storage.
The main advantage of quantum memory is that it can store vast amounts of information in various quantum states. To put this into perspective, 100 bits of conventional storage would accommodate a mere 12.5 bytes of data. However, 100 quantum computing storage bits could contain more states than all the world's hard drives combined, according to Doug Finke of Quantum Computing Report in a presentation at the 2021 Storage Developer Conference.
However, quantum memory storage is nonpersistent. While classical memory -- i.e., RAM -- is also nonpersistent, RAM stores data until a device powers down or reboots. Conversely, quantum memory can only store data for about 100 milliseconds (ms).
It is also impossible to read a quantum state without altering it in the process. Additionally, users can only read the data once. Reading the data causes the quantum bit to release all the states it has stored. At that point, it simply reflects a value of either 0 or 1.
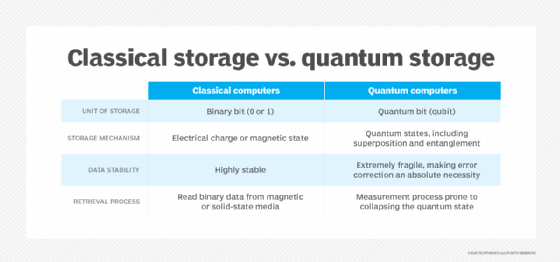
How quantum computers store data
The concept of superposition is often compared to a coin toss. A coin has two sides: Think of heads as being the binary equivalent of 0, while tails represents a binary value of 1. At its completion, the coin toss yields one of these binary values. But while the coin is in the air, it is in a state of neither heads nor tails. Instead, its state is some combination of the two.
Superposition is expressed in a mathematical formula that represents probabilities and amplitudes, while 0 and 1 represent binary states. The equation must be written in such a way that the total probability always equals 1. By using this superposition expression, it is possible to estimate the probability of the state that's being measured as either 0 or 1.
How does quantum memory work?
There are a few ways qubits can store data. Some quantum computers work by storing individual atoms in a vacuum. The atoms are surrounded by an electromagnetic field and stimulated by laser pulses. Data is essentially encoded in the energy level of the electron.
Some quantum computers instead store data by using photons of light. Photons can have both horizontal and vertical polarization. If the horizontal polarization is treated as a binary 0 and vertical polarization as a binary 1, it becomes possible to use either a phase shift -- manipulate the light wave -- or a beam splitter to achieve superposition.
Still other quantum computers use electron spin as a mechanism for storing data. The spin-up state is treated as a binary 0 and the spin-down state acts as a binary 1. A microwave pulse can be used to alter the spin state.
Regardless of which method is used for storing data, quantum states are extremely fragile, making quantum computers highly prone to errors. Such errors can be avoided by applying error correction based on redundancy.
As an example, a single logical qubit might represent several physical quantum bits. Since no single qubit can be trusted to be accurate, multiple qubits can be collectively examined and an inferred value passed to a logical qubit. This process is known as inference and is used to filter out incorrect results.
In physics, there is a concept called the observer effect, which essentially refers to a system being altered as a result of the observation process. To put it another way, you can't measure such a system without affecting it in some way.
This simple principle points to one of the main reasons it is hard to build a quantum computer. In a quantum computer, a quantum state can be lost due to a process called decoherence, which occurs when an environmental factor, such as heat or magnetism, upsets the fragile quantum state. Perhaps even more importantly, the simple act of reading a qubit causes its superposition to collapse into a binary state.
As previously noted, superpositions represent probabilities. A qubit might, for example, have a 70% chance of being equal to 1 and a 30% chance of being equal to 0. Since we can't guess the bit's true value -- there would be a 30% chance of guessing incorrectly -- operations must be run numerous times. This enables the quantum computer to determine with near certainty the true value of the qubit.
The possibilities of quantum computing storage
Since qubits can only store data for a fraction of a second, no quantum hard drives exist.
However, quantum computing is ideal for solving complex mathematical problems, which almost always requires multiple steps; the results of one step serve as input for the next. Qubits can work for such a task as long as the computational processes outpace the rate of data decay. If the technology can read and process data in 50 ms, it doesn't matter that the data essentially evaporates after 100 ms.
Likewise, the idea that users can't read quantum bits without significantly altering them in the process is appealing to security professionals. After all, simply being able to read data in qubit storage guarantees that no one else has accessed the data. Some theorize that it might be possible to build a quantum network that enables data to be sent from point to point with a guarantee that there has been no tampering.
This idea is based on a concept called quantum entanglement. When qubits are entangled, they share identical states -- even if there is a vast distance between them. In fact, collapsing a qubit into its binary state would also cause an entangled qubit to collapse at the same time. Hence, it might eventually be possible to use quantum entanglement to transmit data across great distances in real time.
For example, Voyager 1, a space probe launched in the 1970s, is more than 15 billion miles from Earth. It currently takes radio signals over 22 hours to reach the spacecraft. If a similar spacecraft were designed to take advantage of quantum entanglement, it could theoretically send and receive data in real time.
Quantum memory and quantum computing have many other security implications. For instance, quantum computing will make it possible to break encryption algorithms that are too complex for a classical computer to break. In the case of a ransomware infection, an organization that has access to a quantum computer might be able to decrypt its data without having to pay the ransom. However, a quantum computer might make it possible to create next-generation encryption algorithms that current technology cannot break.
How close are we to a payoff?
Quantum memory has become significantly more reliable in the last few years, but more work is necessary. As of January 2025, IBM had the world's most powerful quantum computer, consisting of 1,121 qubits. In February 2025, however, Microsoft announced its Majorana 1 chip, which Microsoft claims will eventually be able to accommodate a million qubits in a single chip.
Quantum computing is evolving at an extremely fast pace, and it seems likely to become far more practical in the next few years.
Brien Posey is a former 22-time Microsoft MVP and a commercial astronaut candidate. In his more than 30 years in IT, he has served as a lead network engineer for the U.S. Department of Defense and a network administrator for some of the largest insurance companies in America.